Research progress of terahertz super-resolution imaging
terahertz; near field imaging; super lens; photon jet; super resolution reconstruction;convolution calculation
Abstract
Terahertz (THz) imaging technology has recently become one of the most cutting-edge technologies in many fields and has made great progress in its development over the past two decades. With the in creasing demands of scientific research, medical treatment, military and industrial applications, high-resolution THz images have become indispensable. To obtain high-resolution THz images, super-resolution imaging has become a research hotspot. In this paper, the imaging methods of a THz system are reviewed, including continuous wave imaging and pulse wave imaging.
On this basis, THz super-resolution imaging systems and THz signal processing technologies are described in detail. The super-resolution imaging systems include near-field imaging, super lens and terajet effect, etc. The THz signal processing technologies could be grouped as either super-resolution reconstruction and convolution calculations. Finally, the shortcomings of current super-resolution imaging technologies were discussed. There are still some bottlenecks that need to be resolved such as the high manufacturing process requirements of the system, the slow acquisition speed, and the low resolution of the learning samples used to reconstruct images. With this analysis, the research direction of super resolution imaging is proposed.
1 Introduction
Terahertz (THz) waves are the electromagnetic spectrum between millimeter waves and infrared light, with frequencies between 0.1 and 10 THz.
Compared with microwave and infrared imaging, THz wave has the following characteristics: photon energy is low, and has little ionization effect on matter; it is easy to penetrate common non-polar and non-metallic materials such as ceramics and plastics, and can image the interior of them; Molecular vibration and rotational energy levels are in the THz frequency band, which can establish molecular fingerprint characteristics and identify material components; it is highly sensitive to water and is very suitable for water content analysis of materials, etc.
These characteristics make THz technology widely used in fields such as spectrum analysis, safety inspection, medical diagnosis and industrial inspection.
In non-destructive testing, THz testing and imaging technology has been well applied, for example, some research results have been obtained in the testing of thermal barrier coatings. However, in order to study the relationship between the service state of thermal barrier coatings and the internal microstructure, and to intuitively reflect the internal information of the coating, high-quality images need to be reconstructed.
Applications in other fields also have the same requirements. Therefore, how to obtain THz super-resolution images has become a research hotspot in THz technology.
At present, there are two main solutions for THz super-resolution imaging: one is the imaging system, which uses the idea of detecting evanescent waves in the near field to break through the diffraction limit to design related optical devices, collect near-field evanescent waves, and achieve the purpose of super-resolution imaging ; the meta-material-based metalens can amplify the evanescent wave to achieve super-resolution imaging; in addition, the jet effect in the THz band can also achieve super-resolution imaging.
The other is signal processing. Both super-resolution reconstruction and convolution calculation are effective means to improve image quality. Establish a model through learning methods, determine the mapping relationship between low-resolution images and high-resolution images, and further perform super-resolution prediction on unknown low-resolution images to obtain super-resolution images.
Methods such as convolution calculation directly process low-resolution images to improve imaging resolution.
This paper mainly reviews the methods of super-resolution imaging using imaging system and signal processing technology.
2 Terahertz imaging device and imaging principle
2.1 Terahertz imaging device
THz imaging can be divided into continuous wave imaging and pulsed wave imaging.
As shown in Figure 1(a), the THz continuous wave system (Terahertz Continuous Wave, THz-CW) acquires signals and uses the THz wave amplitude information for real-time imaging.
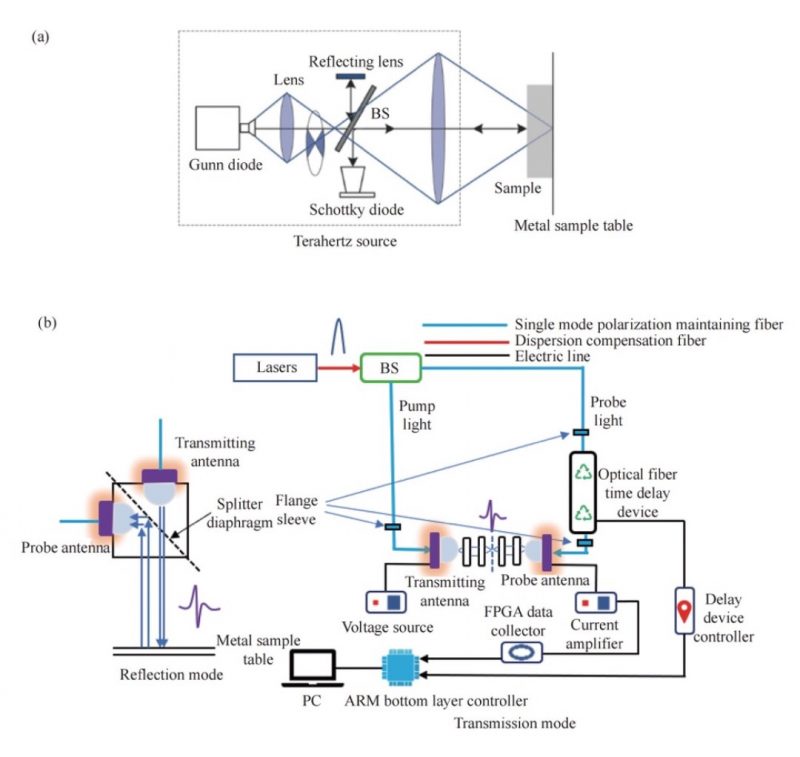
Fig. 1 Schematic diagram of the terahertz imaging device. (a) THz-CW system; (b) THz-TDS system
The Gunn diode is used as the radiation source, and the radiated THz wave passes through the beam splitter (BS) and is focused by the lens onto the sample to be measured, and finally the signal is detected by the Schottky diode and the oscillator.
Figure 1(b) (see the electronic version of the journal for color pictures) is a THz time-domain spectroscopy system (Terahertz Time-Domain Spectroscopy, THz-TDS), which can simultaneously obtain amplitude and phase information for imaging.
The THz-TDS system has two commonly used modes: transmission mode and reflection mode. The THz pulse will carry its information after irradiating the sample. At this time, it will act on the detector together with the detection pulse to realize signal acquisition. According to the equivalent time sampling theorem, the recovery time domain THz wave signal to realize the detection of THz pulse.
2.2 Principle of terahertz imaging
The continuous imaging system applies THz signal intensity information to imaging, and the moving sample is scanned point by point to obtain a two-dimensional image.
At present, some researchers have proposed that the frequency modulation of continuous THz waves based on the triangular wave modulation principle can realize the collection of information at different depths inside the sample.
When a THz pulse is applied to the sample, its transmitted or reflected waveform can be obtained. Among them, the refractive index, absorption coefficient, and thickness of the sample to be measured will change the pulse amplitude and phase of the THz wave.
By translating the sample, THz waveforms at different points can be obtained, thereby constructing a complete THz image of the sample under test pixel by pixel.
Since each pixel contains a complete time-domain waveform, the maximum amplitude, minimum amplitude or arrival time of the THz time-domain waveform can be used to reconstruct a two-dimensional image, which is transformed into the frequency domain by Fourier transform. The amplitude of a single frequency point Or the phase can also be used to reconstruct the image.
THz-TDS tomography images the internal structure of three-dimensional objects. The time-domain waveform amplitude at a specific time point is used as the imaging feature to reconstruct a two-dimensional image, and the three-dimensional image of the measured object can be reconstructed by stacking two-dimensional images at different time points.
The time resolution of THz pulses is on the order of picoseconds, so the time-of-flight method can determine the position of the interface with micron-level resolution, and can also obtain the refractive index information of the material by analyzing the reflectivity at the interface.
3 Terahertz super-resolution imaging system
According to the diffraction effect, in the process of optical imaging, what a point object forms after passing through the optical system is not a point image, which fundamentally limits the imaging performance of the optical system.
The near-field information in the sample scattering information includes the evanescent wave that characterizes the high-frequency components of the sample. Therefore, some researchers have realized near-field imaging by detecting the evanescent wave, breaking through the limitation of the diffraction effect, and improving the imaging resolution.
3.1 Terahertz near-field imaging
The electromagnetic field emitted or scattered by an object can be divided into two parts: the far field and the near field.
Among them, the far field has energy flow propagation, does not carry sample detail information, and the amplitude is inversely proportional to the propagation distance; the near field has no energy flow propagation, but carries more detailed information, and the amplitude decays exponentially with distance.
As a standing wave in the near field, the evanescent wave propagates at the boundary of the medium, as shown in Figure 2(a), and only exists within the wavelength range of the sample surface.
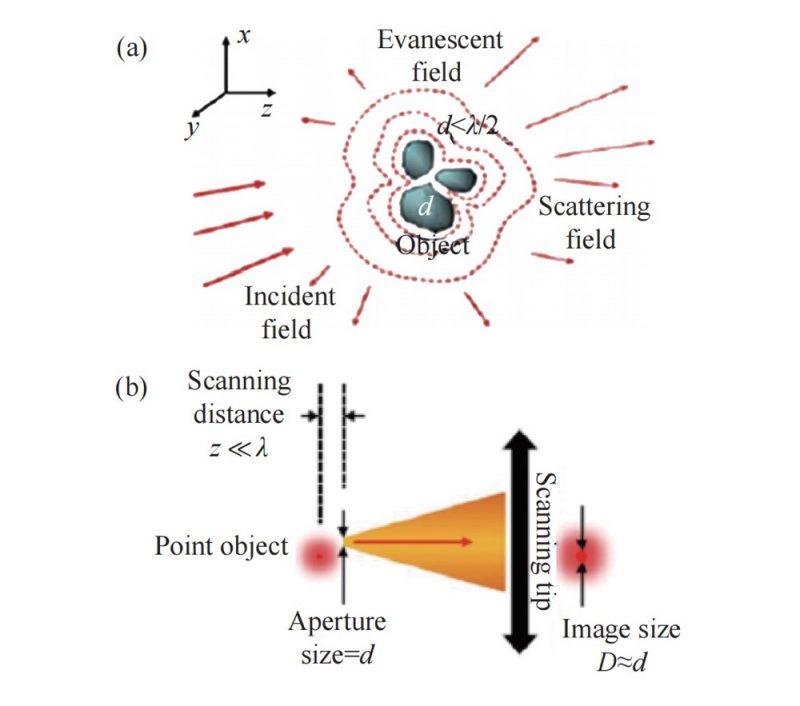
Fig. 2 Schematic diagrams of (a) evanescent field and (b) near field scanning
Synge et al. first proposed the application of a near-field scanning system to achieve optical super-resolution imaging, as shown in Figure 2(b). A resolution of λ/20 can be achieved by probing evanescent waves in the near field with the probe.
Using microwave, infrared, visible light and other mature methods, the researchers proposed a near-field imaging technology in the THz band to achieve non-destructive scanning of the surface of objects.
Figure 3(a) is the confocal optical imaging system, which can initially achieve resolution.
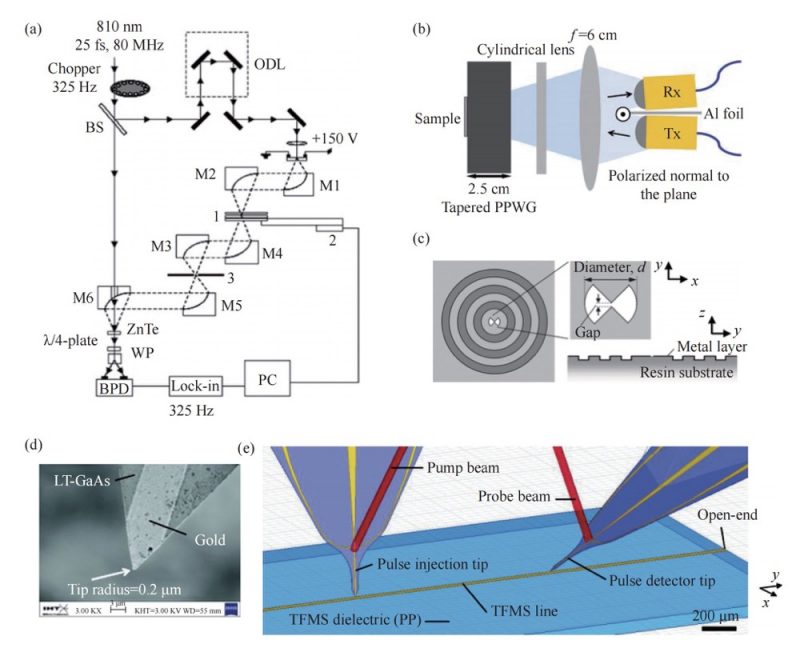
Fig. 3 Principle diagram of Terahertz near field imaging method. (a) Schematic diagram of confocal method; (b) schematic diagram of waveguide system; (c) schematic diagram of aperture system; (d) schematic diagram of photoconductive probe; (e) schematic diagram of photoconductive probe measurement
Subsequently, the researchers found that the use of waveguides to detect reflected signals can reduce transmission loss, improve the coupling efficiency of incident and outgoing THz waves, and further improve imaging resolution.
Figure 3(b) uses the parallel slab waveguide as a coupling enhancement device, and uses the filtered back projection algorithm to reconstruct the image, and the minimum resolution can reach 100 μm (λ/15).
Similarly, Yu et al. combined 3D printing technology to design a THz air-core confocal waveguide to achieve super-resolution imaging.
The aperture rule places the subwavelength aperture in the near-field region, and collects the evanescent wave through the detector, and its spatial resolution is not limited by the incident wavelength. Figure 3(c) shows the subwavelength aperture of the concentric periodic groove. This structure realizes the spatial resolution.
The structure of the photoconductive probe is shown in Figure 3(d). The photoconductive antenna is designed as a sub-wavelength tapered tip structure, and the tip is placed in the near-field region of the sample to obtain ultra-high resolution.
As shown in Figure 3(e), a probe with a diameter of 0.2 μm is used to detect the packaged chip, and the minimum resolution accuracy can reach 0.55 μm. At present, the photoconductive probe measurement technology has been widely used in biomedical diagnosis, circuit defect detection and other related fields. Wide range of applications.
3.2 Terahertz metalens
Pendry et al. found that the amplitude of the evanescent wave increases exponentially when the electromagnetic wave is transmitted in a material with a negative refractive index. Based on this, a “perfect lens” theory was proposed, also known as a super lens, which can amplify the evanescent wave.
However, negative-index materials do not exist in nature.
Metamaterials are typical representatives of subwavelength-scale micro-nano devices, and are macroscopic composite materials composed of sub-wavelength-scale ordered structural units. By designing different sizes and structures, negative or ultrahigh refractive indices can be obtained.
Figure 4(a) is a split resonant ring structure, under the excitation of the magnetic field component perpendicular to the torus, a magnetic response is generated, and its magnetic resonance frequency is about 1.0 THz.
Figure 4(b) (see the electronic version of the journal for color pictures) is a multilayer absorber, which is composed of a metal wire resonator and a lossy dielectric. It can achieve more than 95% high absorption rate.
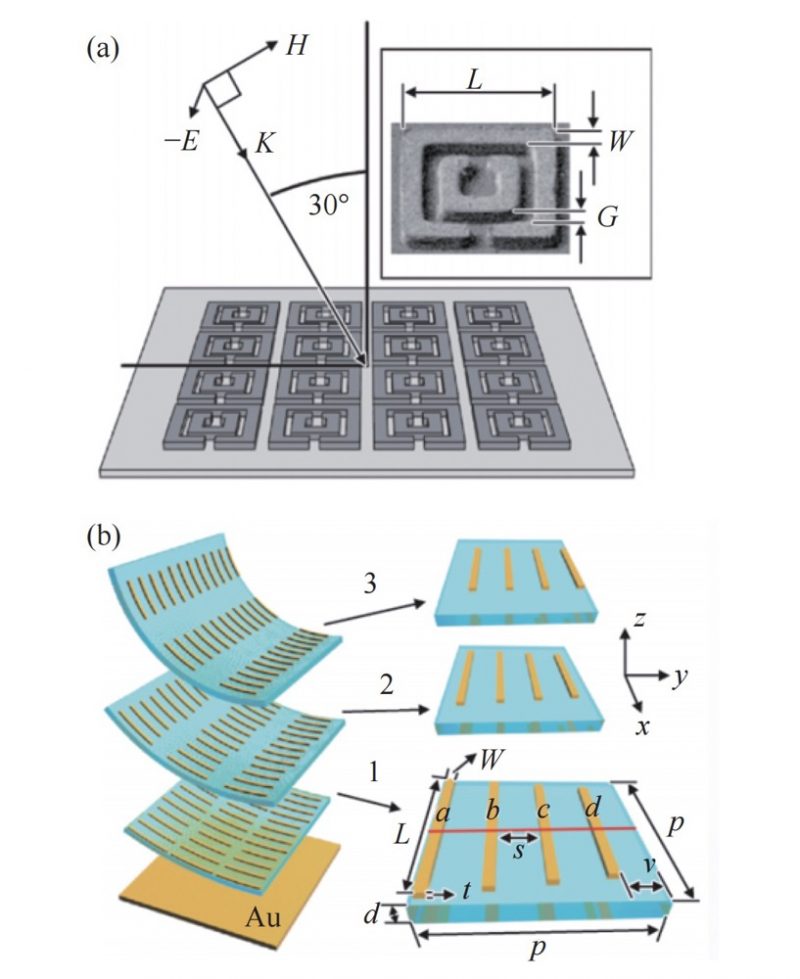
Fig. 4 Terahertz metamaterials. (a) Split resonant ring; (b) terahertz absorber
Grbic used the negative refractive index to carry out imaging experiments, and first obtained the highest imaging resolution in the microwave frequency band.
Due to the serious loss in the working process of the metamaterial, the researchers changed their thinking to develop a “single negative” lens, which can achieve evanescent wave amplification only by making the lens dielectric constant negative.
In the THz frequency band, ideal metalenses can be obtained by using periodic metal structures. The common periodic metal structures mainly include metal gratings and sub-wavelength periodic metal wires.
Metal grating metalens with anisotropic hyperbolic dispersion material properties for channel transport imaging. Figure 5(a) (see the electronic version of the journal for color pictures) is a metal grating designed by Jung et al., which can achieve imaging resolution at a center frequency of 3 THz.
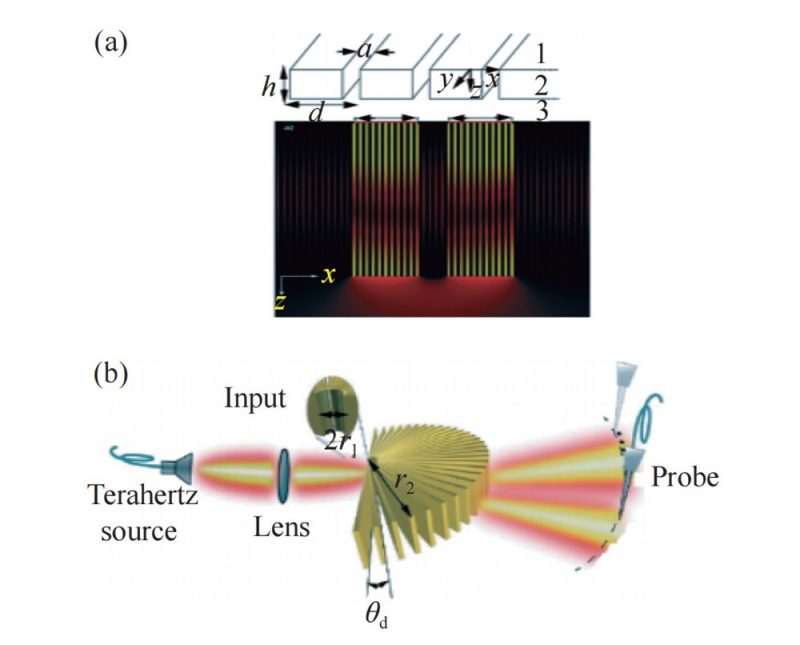
Fig. 5 Terahertz grating metalens. (a) Metal grating metalens; (b) sector grating metalens
Subsequently, Huang et al. improved the above grating structure and used a fan-shaped grating, as shown in Figure 5(b) (see the electronic version of the journal for color pictures), which can extend the imaging distance to the far field and obtain imaging at a center frequency of 0.3 THz resolution.
Subwavelength periodic metallic metalens can transmit and control evanescent waves by adjusting material parameters. Figure 6(a) (see the electronic version of the journal for color pictures) is the first time that Belov et al. used a periodic metal structure to design a metalens, and designed the metal structure to be radial, and the imaging resolution obtained by simulation.
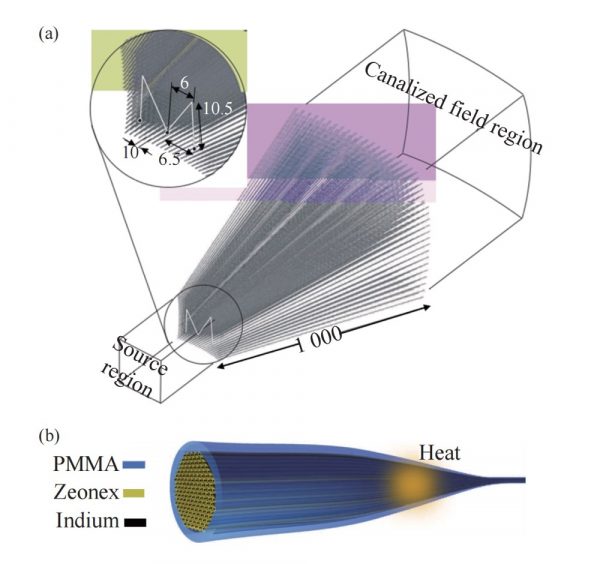
Fig. 6 Terahertz metal metalens. (a) Radial metal wire metalens; (b) periodic metal wire metalens
The obtained resolution was then simulated in the infrared band and verified at a center frequency of 10 THz. The researchers found that the material dispersion and loss characteristics became more prominent as the frequency increased.
Tuniz et al. designed a periodic metal structure as shown in Figure 6(b) (see the electronic version of the journal for color pictures). The surface consists of polymethyl methacrylate (Polymethyl Methacrylate, PMMA) hollow tubes stacked with indium wires (Indium), which are surrounded by cycloolefin polymers (Zeonex).
The absorption rate of polymers in the THz band is low, and the imaging resolution is obtained by near-field scanning of the microwire array at a center frequency of 0.11 THz. For the first time, the super-resolution imaging capability of periodic metal wires has been verified in the THz band. However, the subwavelength periodic metal structure metalens has a large structure and high loss, which still has many inconveniences in practical applications.
Graphene is a single-layer semi-metallic material that is electrically or chemically doped. The working frequency band of graphene surface plasmon (GSP) with different doping methods varies from infrared to THz.
Based on the idea of plasmonic metalens, GSP can realize super-resolution imaging in the THz frequency band. Figure 7(a) (see the electronic version of the journal for color pictures) is a double-layer graphene structure designed by Taubner et al. The resolution can be obtained by identifying the metal slit, and the imaging experiment of the metal circular hole has achieved a resolution of λ/11.
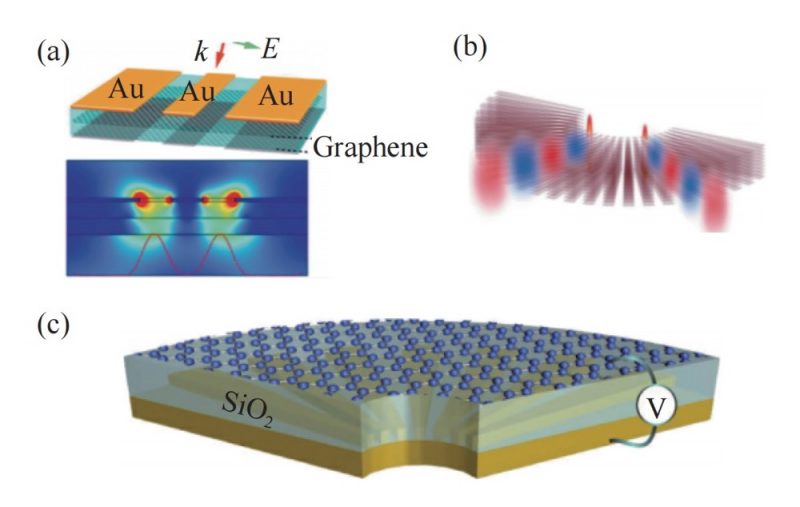
Fig. 7 Terahertz graphene metalens. (a) Dobule-layer graphene metalens; (b) sector multilayer graphene hyperbolic metalens; (c) sector modulated graphene hyperbolic metalens
Unlike optical hyperbolic dispersion materials, graphene hyperbolic dispersion materials can support both transverse electric and transverse magnetic waves.
The fan-shaped metalens designed by Andryieuski et al., as shown in Figure 7(b) (see the electronic version of the journal for color pictures), can achieve far-field super-resolution in the THz band through the angular stacking of graphene and dielectrics.
Subsequently, Tang et al. designed a single-layer graphene superlens combined with fan-shaped modulation. The electrode structure is shown in Figure 7(c) (see the electronic version of the journal for color pictures). THz waves are modulated at a bandwidth of 4.5-9 THz, realizing the Super-resolution imaging.
At this stage, the processing and testing of graphene are difficult to complete, especially the periodic modulation of graphene still has great challenges.
Metasurface is a two-dimensional structural form of metamaterials, which provides a new idea for THz metalens.
Jiang et al. designed an all-dielectric metasurface lens with a focal length and radius of 300 Å, and a maximum incident angle of 48° when converging, proving that the metasurface has focusing and imaging capabilities in the THz band.
Subsequently, Yang et al. combined the optical superoscillation theory to design a metasurface lens, and obtained a focused spot with a full width at half maximum of 0.67, breaking through the diffraction limit.
In summary, metalens imaging can achieve super-resolution reconstruction very well, but most of the current metalens imaging methods are still in the laboratory research stage, and have high requirements for the detection environment, and the problems of loss and integration are still very serious.
3.3 Terahertz jet effect
The researchers found that the dielectric microspheres can obtain imaging resolution beyond the diffraction limit under a white light source.
The microsphere lens can convert evanescent waves into propagating waves, and its high-frequency information can enter the optical system, which can realize super-resolution imaging. The principle of this super-resolution imaging is based on the photon jet effect.
The polytetrafluoroethylene dielectric cylinder is placed at a distance of 0.5 mm (λ/5) from the sample surface, and the incident THz wave is refocused and modulated to realize the sub-wavelength too-jet effect, and the imaging through the THz-CW system reaches λ /2.3 resolution.
As shown in Figure 8 (see the electronic version of the journal for color pictures), a polytetrafluoroethylene dielectric ball with a diameter of 3 mm is placed between the two parabolic mirrors of the THz-TDS system to image a silicon-based dielectric grating with a width of 110 μm , the dielectric grating stripes can be clearly distinguished.
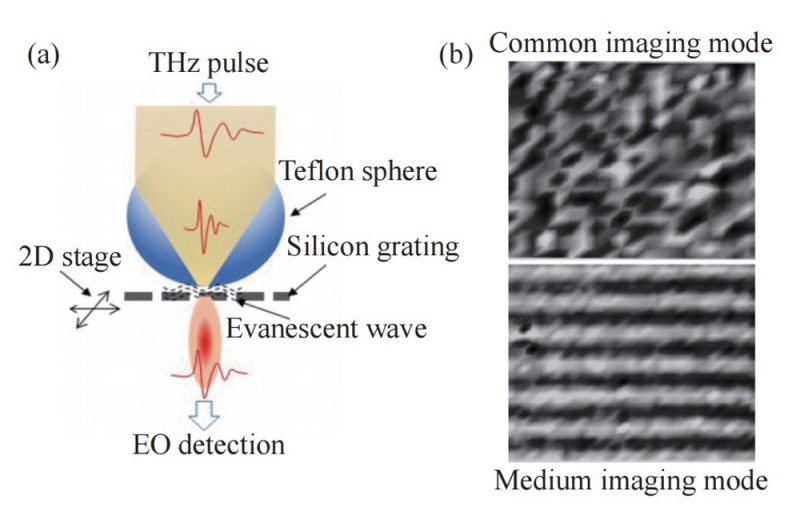
Fig. 8 Terajet THz-TDS application. (a) Working diagram of teflon sphere; (b) comparison chart of silicon dielectric grating imaging
In summary, the too-jet effect can also achieve super-resolution imaging, but there are still the following problems:
(1) The consistency between the shape and size of the light field generated by the THz wave passing through the dielectric structure and the simulation results needs to be verified experimentally;
(2) The enhancement and detection of ultra-fine structure information, and the extraction of super-resolution information also need further research and discussion.
4 Terahertz signal processing technology
In existing THz imaging systems, applying signal processing technology to improve resolution is an economical, practical and effective means. At present, the commonly used methods mainly include image super-resolution reconstruction and convolution.
4.1 Terahertz image super-resolution reconstruction
Image super-resolution reconstruction can be mainly divided into two categories, one is the image interpolation method, which does not require training samples, and improves the image resolution by enhancing the edge of the image; the other is the application learning method to improve the image resolution, in which Sparse coding and convolutional neural networks are currently commonly used learning methods.
Guo et al. used an image interpolation method for THz image super-resolution reconstruction, in which low-frequency information was effectively recovered, but high-frequency information was lost.
In order to better preserve high-frequency information, some researchers combined learning methods with terahertz image super-resolution reconstruction.
Super-resolution reconstruction based on learning methods is widely used in various fields such as optics and electronics.
Deng et al. proposed a locally constrained sparse coding method for super-resolution reconstruction of infrared images. But in this sparse model, the incompleteness of the dictionary limits the effect of super-resolution reconstruction.
Based on this, Shao et al. introduced deep learning methods into super-resolution reconstruction, and used convolutional neural networks to establish training models to achieve super-resolution reconstruction. Similarly, Xi et al. proposed a super-resolution reconstruction method based on a deep residual network. This method is mainly used to solve the problems of large amount of calculation, slow convergence speed and blurred image texture blocks of convolutional neural network.
During the development of infrared image super-resolution reconstruction technology, some scholars have studied the learning-based THz image super-resolution reconstruction. Lu et al. achieved THz image super-resolution reconstruction through a convolutional neural network model.
Among them, the convolutional neural network is trained at a discrete fixed level, which makes the network recovery level not match the THz image, and the reconstruction process is computationally intensive and time-consuming.
Li et al. combined the three-dimensional degradation model of the THz imaging system, and determined the relationship between the imaging range and the corresponding image recovery level through the Point Spread Function (PSF), as shown in Figure 9.
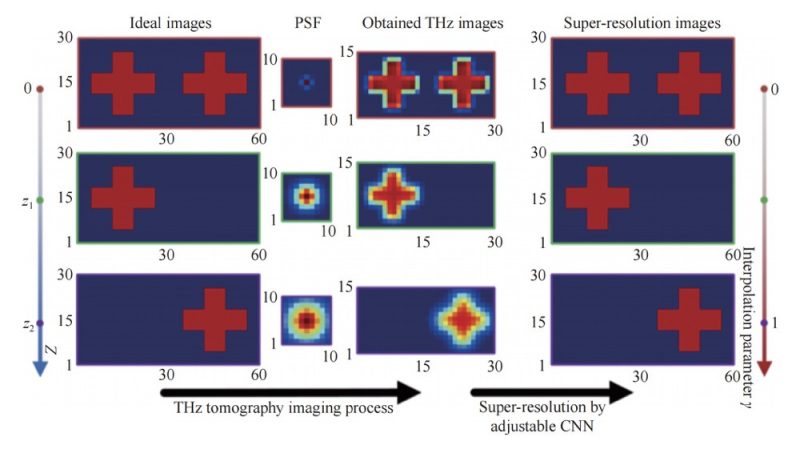
Fig. 9 Schematic diagram of an adaptive super-resolution method
In view of the fact that the PSF at different depths of THz wave focus is constantly changing, the relationship between the imaging range and the corresponding image restoration level is determined by the model, and the super-resolution reconstruction of THz images at different depths is realized.
4.2 Application of convolution calculation in terahertz imaging
Longitudinal resolution is also an important indicator for evaluating imaging quality. The current methods to improve longitudinal resolution mainly include wavelet analysis and deconvolution, both of which perform THz signal processing through convolution operations. Wavelet analysis has played an important role in many fields such as signal processing, data compression, image processing, seismic exploration, speech processing, and mechanical fault diagnosis.
The absorption of water vapor on THz waves will cause signal interference and reduce the signal quality. Deng et al proposed to use the Gabor wavelet analysis method to process THz signals, and the results show that wavelet transform is suitable for the time-domain characteristic analysis of THz waves.
Subsequently, Chen et al. used the THz-TDS system to scan and image the four samples of starch, aspirin, soda and benzophenone point by point in the air, and processed the THz signal by wavelet transform, and the sample image recognition effect was clear.
In the detection of complex and thin samples, the THz center wavelength is on the submillimeter level, but the longitudinal resolution is difficult to exceed this level, and the internal interface of the sample cannot be distinguished.
Dai et al. used an imaging system with a center frequency of 0.3 THz to detect thin samples of multi-layer thermal insulation felt with embedded defects. The distance between the reflection peaks on the upper and lower surfaces was too close to cause aliasing, and Gaus2 wavelet was used to process the signals of all detection points , which can effectively resolve the aliasing peaks.
Figure 10 (see the electronic version of the journal for color pictures) shows the image comparison before and after wavelet transform processing.
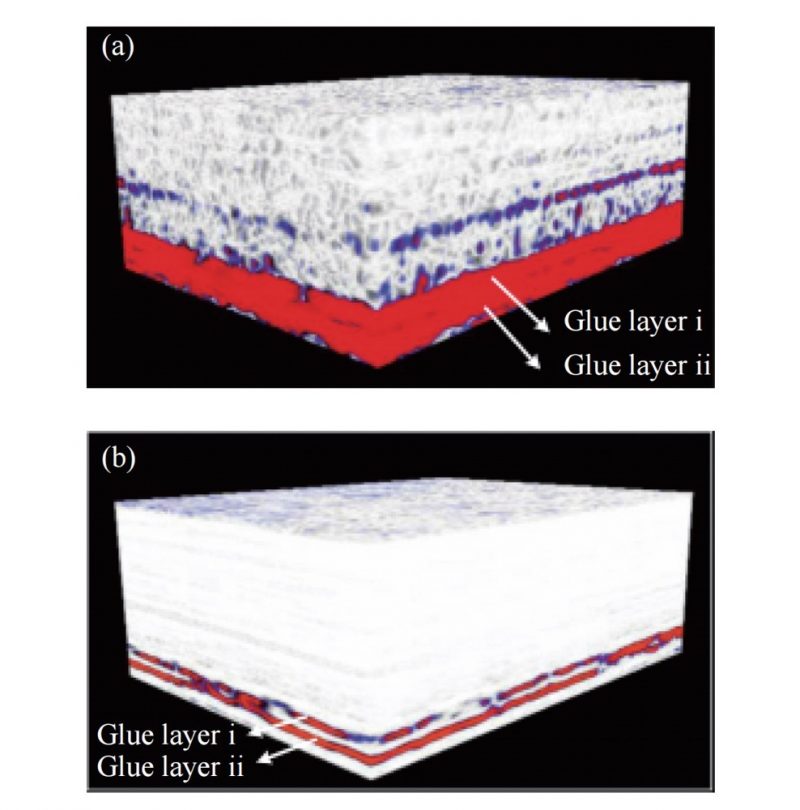
Fig. 10 Imaging comparison diagram (a) before and (b) after Gaus2 wavelet transform
It can be seen that after wavelet transform processing, the defect area of the heat insulation felt can be accurately located, and it is in good agreement with the actual sample.
Zhang et al. used a THz imaging system to detect integrated circuits, and selected db5 wavelet base for processing, which improved the imaging resolution. Similarly, Zhang et al. used a THz imaging system to detect prefabricated wedge-shaped defective phenolic plastic plates, and used wavelet transform soft and hard thresholds to process the detection signals before imaging.
The results show that this method can clearly distinguish the structural changes inside the sample, which effectively improves the imaging resolution.
Using THz deconvolution can also solve reflection peak aliasing, resulting in improved longitudinal resolution.
Dong et al. used an imaging system with a center frequency of 1.5 THz to detect polyester coatings. Various failures such as corrosion, delamination, and air bubbles were pre-fabricated in the coatings, resulting in serious aliasing of the obtained THz signals. After deconvolution, the reflection peaks are easier to identify, so the imaging resolution can be improved.
Similarly, Ye et al. applied a THz imaging system to detect thermal barrier coatings, and prefabricated two parallel cracks with different specifications inside the coating. By performing deconvolution processing on the THz signal, the crack width can be effectively resolved, and the minimum resolution accuracy is increased from 130 μm to 80 μm.
However, the convolution method eliminates the aliasing phenomenon by compressing the reflection peak pulse width, which will cause distortion of the THz time domain signal during the compression process, making the thickness measurement result inaccurate.
Therefore, when using deconvolution, it is necessary to determine the parameters such as the refractive index of the sample and the extinction coefficient in advance in order to accurately improve the longitudinal resolution.
5 Conclusion
THz super-resolution imaging is an eternal goal pursued by imaging systems.
In order to improve the imaging resolution, researchers have devoted a lot of energy to the development of imaging systems and signal processing.
Future super-resolution imaging research directions mainly include the following aspects:
(1) Due to its compact structure and high power, THz quantum cascade lasers have attracted much attention in the field of THz imaging, and high-power THz sources are one of the keys to improving the stability of imaging systems. Therefore, combining THz quantum cascade laser technology for real-time The research and development of large depth of field and super-resolution imaging systems are the focus of attention in various fields;
(2) The fusion of programmable technology and metamaterials is currently a hot spot. At present, intelligent real-time imaging has been realized at a working frequency of 3 GHz. Therefore, it is expected to further move towards the THz frequency band;
(3) The super-resolution image reconstruction algorithm is a cutting-edge topic. Introduce the AI algorithm in the THz band to build a model, establish the mapping relationship between conventional imaging and THz imaging information, and realize super-resolution reconstruction.
All in all, the improvement of imaging resolution is a complex issue that requires research and breakthroughs in various aspects such as THz source, optical system, and signal processing.